1.2 Multidisciplinary Engineering Design
Much of engineering design and development is accomplished by teams comprising engineers from multiple disciplines. Consider a smart phone: a relatively small package stuffed with electronic circuitry, a power supply, several user interfaces (screen, microphone, speakers, buttons), all packaged within a housing made of material that is lightweight yet usually strong enough to withstand being dropped on a concrete floor, while also supporting the function of an antenna that needs to connect the electronics inside to the free space outside the phone. The user interfaces and the size, weight, and design of the phone need to incorporate human factors as well as customer buying and using tastes into the design. The device needs to radiate enough power to enable it to communicate with cellular base stations yet not so much power that it poses a hazard when the phone is placed near the user’s ear. The graphic display needs to be large enough, and with high enough resolution, to enable the user to view movies and other media at arm’s length, yet not so large and bright that it drains the battery too quickly. Engineers having expertise in electrical, computer, materials, human factors, and manufacturing, and other disciplines need to be involved in the design of such a device. As another example, consider an artificial pacemaker: an electronic device surgically placed under the skin of a patient’s chest to help regulate heart rate. A small microcontroller interprets cardiac signals from electrodes placed within the chest; when an abnormal beat-to-beat heart rate is detected, the device generates short, low-voltage pulses that stimulate the chambers of the heart, causing it to beat at a normal rate. The pacemaker, containing the microcontroller, battery and voltage pulse generator needs to be designed in such a way that it can reside within the body for 10 years or longer. Developing such a device draws on expertise from biomedical, electrical, computer, and mechanical engineering disciplines, among others. Hearing aids, endoscopes, PillCams, new artificial retinas and robot limbs, smart cars, robots, renewable energy systems, drone-based inventory and delivery systems, spacecraft, smart watches, exercise machines, entertainment systems, computers, robotic vacuum cleaners, laptops, microwave ovens, wireless earbuds, automobiles, aircraft, weather radars, lighting systems, smart coffee pots, etc… are all examples of products and technologies that rely on multidisciplinary engineering design.
The point of these examples is to illustrate that many of the products, technologies, and systems that we commonly use and rely upon are brought about by multidisciplinary collaboration among engineers of different disciplines. A mechanical engineer designing the packaging, the thermal management, or the power supply needs to effectively communicate and collaborate with the electrical engineer designing the generator or the computer so that the resulting system works efficiently and durably. The biomedical engineer’s knowledge of biology, medicine, and engineering needs to be brought into collaboration with the electrical engineer to produce a system that will make contact with body tissues or control functions within the body. Industrial and systems engineers provide planning and structure to the design & development enterprise. Their process expertise guides the team from initial concept, through requirements development, to architectural trade offs and design studies, to subsystem design, integration and testing, and then ultimately, deployment and support of the product or system in the world.
Engineers obviously need to be knowledgeable and skilled within their particular disciplines in order to contribute. At the same time, engineers need to have sufficient shared vocabulary as well as concept and device knowledge of each other’s discipline to effectively work in multidisciplined design, development, and manufacturing teams. Engineering design involves trade-offs and give-and-take to realize a design that meets requirements and constraints. The constraints and trade-offs suggested above, namely, display brightness vs. battery life; device performance vs. functionality; package size vs. the need to be swallowed by a person or otherwise reside in the body; durability vs. cost and aesthetic appeal; complexity vs. manufacturability & serviceability are but a few of the many facets of the trade-space that defines engineering.
Engineering activities include establishing and maintaining requirements; designing on paper; modeling and simulating on a computer and the lab bench; prototyping; re-designing; component selection; manufacturing; and other activities. Multidisciplinary engineering collaboration involves communicating, explaining, justifying, debating, balancing, trading, and other activities. Respecting – but not fearing – the other discipline is necessary. Consider the ventilator problems discussed at the beginning of this chapter: the ICU ventilators must work correctly and reliably with the design modifications in place; if they fail, a patient may die. It isn’t always life and death, but there are always consequences of bad engineering and ineffective engineering collaboration. Understanding and respecting – but not fearing – the underlying engineering science and methodology is important: this is what enables engineering functions to be carried out.
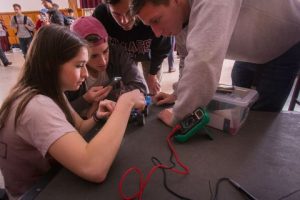