2 Basics of Matter
A Deeper Structure of the Atom
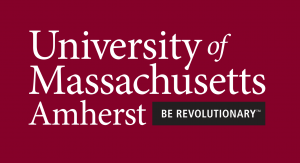
Instructor’s Note
The things you need to know for your homework and quizzes are:
- Electrons and protons are charged, neutrons are not; the size of the charge on the electron and proton is the same, but the signs are different, so same magnitude different sign
- Opposites attract and that is what holds the atom together
- Protons and neutrons have the same mass, and electrons are way lighter
- Protons and neutrons made of stuff, electrons are fundamental
- The nucleus is super tiny relative to atom
You should be familiar with the basic structure of the atom, but as a review, in the middle of the atom the positively-charged protons and neutrons are huddled together in the nucleus.

However, you might not be familiar with the related symbols. This symbol, means proton, p for proton, and then plus to remind us that it has a positive electrical charge (If you’re wondering, can you have a negatively charged proton? Yes, it’s called an proton, see below.) You also have neutrons. Neutron has zero charge, so it’s symbol is
. Those are huddled together in the nucleus.
Surrounding the nucleus is a big cloud of negatively charged electrons, so we will use the symbol for electron. It’s the attraction between the positively charged protons and the negatively charged electrons that sort of hold the entire atom together, and how that all works will be the emphasis of Unit III.
Electrons are a big focus of this course, so it is worth discussing what they are made of. To our best of our knowledge they are not made up of anything. They are fundamental, we have been trying to smash them apart, but no luck. Maybe it’s possible, but no one’s been able to do it. If it is possible, we haven’t hit it hard enough. That’s very much the particle physics approach to everything- hit it harder and see if it breaks. So, electrons are fundamental building blocks- as far as we know they’re not made up of anything.
Protons and neutrons on the other hand, are a lot more fun, because they are made up of smaller pieces called quarks. There are six kinds of quarks, we have ‘up’, ‘down’, ‘strange’, ‘charm’, ‘top’, and ‘bottom’. Those are their official scientific names, I kid you not. In Figure 7 below, the sizing of the circles shows you the masses, how heavy this stuff is (but not their sizes – as far as we know the quarks have zero size!). The ‘top’ quark, the heaviest of the known quarks, actually has about the same mass as an entire atom. It’s quite a heavy little thing. Three of these quarks, ‘top’, ‘bottom’, and ‘charm’, are actually heavier than protons.
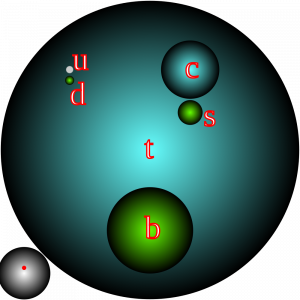
But, if charms, bottoms, and tops are all heavier than protons and neutrons, so what makes up a proton in a neutron? Protons and neutrons are made up of just these two ‘ups’ and ‘downs’. So, a proton is made up of two ‘up’ quarks and one ‘down’ quark, a neutron on the other hand is two ‘down’ quarks and an ‘up’ quark as shown below in Figure 8.

Now you start doing math, so you need the proton to have +1 charge, the neutron to have 0 charge, you have two ‘ups’ and a ‘down’, and two ‘downs’ and an ‘up’. If you play with those numbers, what do you get? You have that ‘up’ quarks have a charge of 2/3 that of the proton, and down quarks are -1/3 that of the proton. And this works out: think ‘up’ ‘up’ ‘down’ so that’s: (+2/3) + (+2/3) + (-1/3) = +1, which is the charge of a proton, and it works out.
Similarly, for the neutron think, (-1/3) + (-1/3) + (+2/3) = 0: the charge of a neutron, they work out.
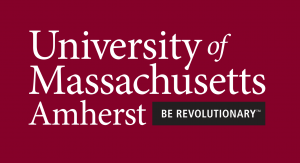
Instructor’s Note
What’s your big takeaway for this? Electrons are fundamental to our knowledge and cannot be broken apart. Protons and neutrons are made up of smaller stuff.
The other key things to know about atoms: protons and neutrons are very very close to the same mass, but neutrons are a tiny bit heavier, but not by much. Electrons on the other hand are way lighter than protons or neutrons. In fact, the electron is the lightest known particle to have electric charge with a mass of . Protons on the other hand, are much bigger,
.
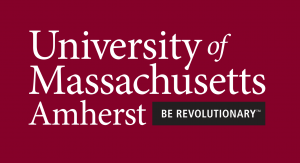
Instructor’s Note
What should you take away from this? Protons are way more massive than electrons, roughly 2,000 times (1836 times to be specific).
If you prefer to think about atoms instead of protons and neutrons, you can think about a Helium atom, you know there are two protons, two neutrons, and two electrons. The electrons make up 0.03% of the mass of helium. Electrons don’t weigh squat. They don’t really matter as far as mass goes. While the nucleus has most of the mass, it doesn’t take up a lot of space. The standard analogy that people make is if you blow up the atom to the size of a large college football stadium, bigger than ours, the nucleus is roughly the size of a marble. Atoms are a whole bunch of empty nothing. The nucleus is about the size of a pea but it is 99.97% of the mass is in that marble comparatively speaking.
Conservation of Mass is a lie! Conservation of Energy and Conservation of Charge are true! How this is connected to antimatter.
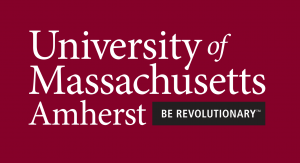
Instructor’s Note
We will be occasionally dealing with antimatter in this class. You need to know that matter and antimatter are identical in mass, but opposite in charge: an anti-electron has a positive charge. You also need to know that when matter and anti-matter come together the result is pure energy.
In chemistry, mass is never created or destroyed. This is often described as the conservation of mass. However, this principle is actually NOT universally true! In more exotic situations, such as in particle accelerators, mass, , can be created from energy in the amount using Einstein’s famous relation:
This famous equation basically says that mass is just a particular form of energy: a highly concentrated form as even a small amount of mass, when multiplied by , yields a large amount of energy. In fact this is the energy you see everyday as sunlight as will be explored in your homework!
Problem 2: How much energy is produced in the sun from converting 2 protons and two neutrons into a helium nucleus?
In all observations to this point, every time a particle is created in this way another, having the exact same mass but opposite charge is always created along with it. The two particles are “matter-” counterparts. For example, an anti-electron would usually be created at the same time as an electron. The anti-electron has a positive charge (it is also called a positron as in positron emission tomography). Since the electron is negatively charged and the positron is positively charged, the total charge created is zero. This is a manifestation of one of the most important Laws of the Universe: Conservation of Electric Charge. Consider the reaction in Figure 4a: energy (with zero net charge produces an electron and a positron (also zero net charge!).
The reverse is also true: when matter and antimatter counterparts are brought together, they completely annihilate one another as seen in Figure 4b. By annihilate, we mean that the mass of the two particles is converted to energy E, again obeying the relationship
Again, the total electric charge is conserved: we began with zero net charge (one negatively charged electron and one positively charged positron) and the result was energy with zero net electric charge.

All particles have antiparticle counterparts: there are negatively charged anti-protons made up of two anti-up quarks (charge -2/3) and one anti-down quark (+1/3). Similarly, there are even anti-neutrons made up of two anti-downs and one anti-up (note the anti-neutron also has charge zero, but would still annihilate if it met a regular neutron!).
Making Connections: Conservation Laws
Only a limited number of physical quantities are universally conserved. Charge is one—energy, momentum, and angular momentum are others. Because they are conserved, these physical quantities are used to explain more phenomena and form more connections than other, less basic quantities. We find that conserved quantities give us great insight into the rules followed by nature and hints to the organization of nature. Discoveries of conservation laws have led to further discoveries, such as the weak nuclear force and the quark substructure of protons and other particles.
The law of conservation of charge is absolute—it has never been observed to be violated. Charge, then, is a special physical quantity, joining a very short list of other quantities in nature that are always conserved. Other conserved quantities include energy, momentum, and angular momentum.
Problem 3: How much energy is released when an electron and an anti-electron (positron) annihilate during positron emission tomography?
Where is all the anti-matter?
If every time an electron is produced, I also get a positron, why do we see electrons everywhere while positrons are so rare? In fact, why do we exist at all? In the early Universe after the Big Bang, there should have been equal amounts of matter and anti-matter which should have then annihilated itself leaving a Universe with no matter – just energy!
This is a good question! In fact, it is a question to which we do not know the answer! It may surprise you to learn that we don’t know the answer to something as fundamental as “why is there something instead of nothing?” This is the fun of science! It means that there is still work to do!
For each type of particle in the universe, there is an antimatter counterpart. These antimatter counterparts have the same mass as the usual particles, but the sign of the electric charge is reversed: anti-electrons are positively charged, while anti-protons are negatively charged. When matter and antimatter are brought into contact, the result is their mutual destruction (or annihilation) into pure energy.