8 Using Data on Student Values and Experiences to Engage Faculty Members in Discussions on Improving Teaching
Gili Marbach-Ad, Carly Hunt, and Katerina V. Thompson
1 Introduction
There is a consensus among employers (Hart Research Associates, 2015, 2018), policymakers (e.g., AAMC-HHMI, 2009; AAAS, 2011, 2015; Heron et al., 2017; PCAST, 2012) and education researchers (Hora, 2016; Matusovich et al., 2014) that undergraduate students need to develop skills such as collaboration, communication, and problem solving. These skills have been variously referred to as twenty-first-century skills (Jang, 2016), soft skills (Finch et al., 2013), and employability skills (Rosenberg et al., 2012). In addition to these widely applicable skills, STEM fields also rely on skills that are somewhat general but not unique to a specific STEM discipline (e.g., computation, data interpretation). We refer to the entire suite of general and STEM-specific skills as cross-disciplinary skills. Cross-disciplinary skills are best developed using evidence-based teaching approaches that actively engage students in learning, such as having students work in groups. However, faculty often rely on traditional lecturing methods, which is at odds with stakeholder recommendations and ineffective in helping students hone these skills (Bok, 2006; Handelsman et al., 2004; Kezar, 2001; Seymour, 2001).
We describe here a strategy employed by the College of Computer, Mathematical, and Natural Sciences (CMNS) Teaching and Learning Center (TLC) at the University of Maryland to help shift our faculty members’ teaching approaches towards practices more effective for building cross-disciplinary skills. Faculty members at research universities identify primarily as researchers, who regularly collect, interpret, and make decisions based on data (Anderson et al., 2011; Brownell & Tanner, 2012; Robert & Carlsen, 2017; Savker & Lokere, 2010). We are using this deeply-rooted professional identity as a lever to design professional development activities that motivate faculty members to reconsider their beliefs, values, and behaviors towards teaching. Our activities were designed not only to relate to the faculty members’ identities as researchers, but also to their departmental and disciplinary identities.
2 Theoretical Background
2.1 Faculty Member Identity and Social Network Membership
We conceptualize faculty members as having individual professional identities as well as identities as members of disciplinary and departmental communities. Regarding individual professional identity, faculty members begin a socialization process in their doctoral programs where they are often encouraged to prioritize research over teaching (Fairweather, 2008). Austin (2011) explains that doctoral education is an “apprenticeship experience” where doctoral students adopt the values and beliefs of their research mentors. They receive implicit and explicit messages from their mentors that they should avoid allocating too much time towards teaching, that teaching detracts from research, and that teaching excellence will be rewarded to a lesser degree than research productivity. These formative experiences result in faculty members identifying as researchers first and as instructors second, which impacts the decisions they make about allocating their time (Brownell & Tanner, 2012; Robert & Carlsen, 2017; Savker & Lokere, 2010) and has direct implications for teaching professional development (TPD). To effectively address issues of identity in TPD, some authors (Robert & Carlsen, 2017) have suggested that TPD activities be designed to respect faculty members’ identities as researchers. Specifically, they propose that interventions that integrate teaching and research could be a point of entry in engaging research-oriented professors more deeply in conversations about teaching.
Faculty members also belong to departments and disciplines (Kezar, 2014; Quardokus & Henderson, 2015; Robert & Carlsen, 2017), which are social systems with unique characteristics (Donald, 1997; Hativa, 1995; Smart & Ethinton, 1995). Therefore, it is critical that teaching and learning reform efforts and professional development activities be sensitive to disciplinary and departmental contexts (Austin, 2011; Bradforth et al., 2015; Coppola & Krajcik, 2013, 2014; Henderson & Dancy, 2011; Quardokus & Henderson, 2015; Wieman et al., 2010). Furthermore, education policymakers have emphasized that university-wide changes in teaching “are sustainable only if combined with changes at the colleges (subsets of universities) and departmental levels that foster a team culture of continuous teaching improvement” (Bradforth et al., 2015, p. 284). They term this the “middle out” approach, where change efforts are situated in colleges and departments. This is distinct from approaches that are “bottom up” (i.e., change instigated by the faculty members who provide instruction to students) or “top down” (i.e., change instigated by senior university administrators). Middle out change initiatives are seen as more likely to be successful and sustained because of their ability to exert influence at all levels of institutional structure (i.e., individual, departmental, and administrative). Consistent with this perspective, there are change theories in the literature that provide rationales for intervening on the department level as well as on the individual level. We elaborate on these theoretical perspectives below and describe how they informed our TPD activities. Additionally, please note that Andrews et al. (this volume) provide another example of a departmental-level approach to change.
2.2 Theory of Change
Change theories have implications for the intervention efforts of change agents (Andrews et al., 2016; National Research Council, 2012), such as TLCs and discipline-based education researchers (DBERs). To guide our efforts, we drew on cultural and social cognitive perspectives of institutional change (Kezar, 2013, 2014). The cultural perspective on change asserts that organizations (e.g., departments) hold unique norms, values, and beliefs that influence individuals within the culture. By implication, change ensues from shifts in department-level values and beliefs. Change agents like DBERs and TLCs must know the current values of a department and align their interventions with those values (Corbo et al., 2016). The social cognitive perspective on change emphasizes the role of individual values and beliefs in guiding behavior, as well as the role of thought processes (Bandura, 1997; Pajares, 1992). The social cognitive perspective asserts that one way change agents can shift beliefs is to introduce new information that will encourage individuals to confront and modify their prior beliefs.
Here we describe our efforts to promote a shift in faculty member beliefs that could ultimately increase their likelihood of adopting evidence-based teaching practices. Building on social cognitive and cultural perspectives on change, as well as theories of professional development and change in K-12 teachers (Desimone, 2009), we designed and implemented a novel approach to engage faculty members in discussions about teaching. Specifically, we presented faculty members with data on their own students’ values, in essence providing them with “new information” that might encourage them to confront their prior beliefs about teaching. We did this during regularly scheduled faculty meetings to promote departmental-level discussion and consensus building. We anticipate that continuing conversations of this type could motivate changes in teaching practices that, in turn, could ultimately impact students’ beliefs about cross-disciplinary skills. We plan to assess these downstream outcomes (i.e., changes in teaching practices and student beliefs) in future research.
3 Methods
3.1 Institutional Context
University of Maryland, a research-intensive public university, enrolls about 27,000 undergraduates. Our TLC serves 321 tenure-track and professional-track faculty members from 10 CMNS departments: Astronomy (ASTR), Atmospheric and Oceanic Sciences (AOSC), Biology (BIOL), Cell Biology and Molecular Genetics (CBMG), Chemistry and Biochemistry (CHEM), Computer Science (CMSC), Entomology (ENTM), Geology (GEOL), Mathematics (MATH), and Physics (PHYS). These departments collectively enroll over 6,000 students.
3.2 Faculty Professional Development in Teaching
During the 2016–2017 academic year, we visited regular faculty meetings in the 10 departments to initiate conversations about teaching. We asked the chairs to allow at least 45 min for our visit. In each faculty meeting, we 1) surveyed faculty regarding their educational values, 2) explained the ways in which the TLC could support their teaching endeavors, 3) engaged faculty in a discussion of student educational values, and 4) sought their insight into observed patterns of student values and how those values corresponded to faculty values.
At the beginning of each meeting, faculty were asked to complete a modified version of the Survey of Teaching Beliefs and Practices for Undergraduates (STEP-U) (Marbach-Ad et al., 2019), which focused on 17 cross-disciplinary skills (e.g., scientific writing, problem solving, working in groups). They first rated how much they valued each skill as an outcome of undergraduate education in their discipline on a scale of 1 (not important) to 5 (very important). Using the same scale, they were then asked to predict how much graduating students in their discipline would value each skill. Finally, we asked them to list any skills important to graduates in their discipline that were omitted from the survey. Surveys were anonymous and collected at the end of the meeting for subsequent analysis.
We next summarized the various professional development activities offered to faculty by the TLC (e.g., individual consultations, opportunities to join faculty learning communities, assistance with disseminating innovative teaching practices). These services were summarized on a handout (Appendix 1) given to faculty for future reference.
To stimulate discussion, we distributed a two-page handout summarizing student survey responses (N=2404) collected from University of Maryland students over five years (Marbach-Ad et al., 2019). Student data were presented in tabular form, along with a summary of information gleaned from student interviews and a statistical analysis[1] of demographic and experiential predictors of student values (see example, Appendix 2). One table (Table A1) showed student response rates and demographics (i.e., gender, underrepresented minority group membership, undergraduate research experience, cumulative GPA, career aspirations). A second table (Table A2) consisted of a heat map showing the percentages of students in each CMNS major that indicated a given STEP-U skill was “important” or “very important” to acquire during their undergraduate studies. The heat map format enabled faculty to make quick comparisons of the degree to which their own students valued particular skills relative to other skills and relative to students in other CMNS disciplines.
After giving faculty a few moments to look over the summarized data, we asked them to suggest possible reasons for 1) discrepancies between their students’ values and their own, 2) the possible relationship between student values and classroom experiences, and 3) discrepancies between their students’ values and those of students from other disciplines. Finally, we engaged them in discussions of teaching strategies that could potentially address the observed discrepancies. These discussions were audio recorded for subsequent analysis.
3.3 Analysis of Faculty Data
Surveys administered during the 10 departmental faculty meetings resulted in 184 responses [ASTR (n=13), AOSC (n=11), BIOL (n=16), CBMG (n=25), CHEM (n = 30), CMSC (n=14), ENTM (n=11), GEOL (n=15), MATH (n = 7), PHYS (n=42)]. We analyzed faculty member survey data in a manner similar to student data by calculating proportions of respondents rating the item as “important” or “very important,” then summarizing the results using heat maps. Transcripts of faculty discussions were reviewed independently by two researchers, who recorded major themes, then discussed them until reaching approximately 90% agreement.
4 Findings
We report on the qualitative and quantitative data from the TPD intervention, as well as our own subjective impressions of the process, to inform future discipline-specific TPD efforts. We summarize major themes in Table 1 and describe below the planning process, general impressions of the faculty discussions, the faculty’s specific insights into factors influencing student values, and their suggestions for improving the survey.
Department | Skills discussed | Faculty insight and reactions to student perspectives | Major discussion points for teaching improvement |
ASTR | Scientific writing, working in groups, application to everyday life, dynamic nature of science | Most students are double majors (most often with physics or mathematics). Faculty were disappointed with students’ lack of appreciation for scientific writing and how much science applies to everyday life. | Working in groups Faculty noted that they had only recently implemented more group work in introductory classes, so the seniors who responded to the survey did not have the benefit of these improvements. |
AOSC | Scientific writing, working in groups, communication, quantitative reasoning | Every undergraduate does a research-based senior thesis, which provides unique opportunities for cross-disciplinary skill development. Faculty suggested that AOSC is an applied science and many students go straight into the workplace after graduation, so it is important to incorporate group work in classes. Faculty were concerned that their students were not valuing quantitative reasoning. | Communication Faculty suggested this could be developed by encouraging students to go to conferences to present undergraduate research. Quantitative reasoning Faculty thought students would benefit from having more assignments that required the application of quantitative reasoning. |
BIOL | Scientific writing, memorization, decision-making based on evidence | Many faculty members expressed surprise that students did not value scientific writing. | Scientific writing Faculty discussed the need to implement more activities to promote scientific writing and the challenges in doing so. |
CBMG | Scientific writing, working in groups, memorization, interdisciplinary nature of science, application to everyday life, communication | Students with different career aspirations have differing values (e.g., pre-medical students have lower appreciation for scientific writing compared to students who are planning to enter graduate school). | Working in groups There was a robust discussion of experiences with group work and ways to implement it more effectively. Memorization Faculty expressed frustration with how memorization has been devalued in recent years. |
CHEM | Scientific writing, working in groups, interdisciplinary nature of science | Faculty underestimated the degree to which students valued many cross-disciplinary skills, and were pleasantly surprised when student values were consistent with faculty values (e.g., scientific writing). | Scientific writing Faculty stressed the importance of incorporating writing assignments throughout the curriculum. Working in groups Faculty discussed strategies for reducing student resistance (e.g., assigning grades individually, rather than assigning the entire group the same grade). |
CMSC | Working in groups | Students do not see themselves as being science majors, so the survey language (geared towards natural sciences) may have seemed inapplicable to their experience. Computer science is an applied science and many students go straight into the workplace after graduation, so it is important to incorporate group work in classes. |
Working in groups This was mainly incorporated into upper-level courses or done outside of classes (e.g., hackathons). |
ENTM | Scientific writing, working in groups | Faculty members discussed lower-level (i.e., first- and second-year) students separately from upper-level students. Students in introductory courses expect professors to provide facts, rather than challenging students to think critically. They perceived that upper-level students were more open to group work. Faculty were frustrated with inadequacies in students’ scientific writing skills. | Scientific writing Faculty attempted to explain differences between disciplines regarding this skill, e.g. biology standards do not emphasize this skill as explicitly as the American Chemical Society recommendations. Working in groups Conversation centered on the importance of working in groups and ways to implement group work more effectively in classes. |
GEOL | Scientific writing | Every undergraduate does a research-based senior thesis, which provides unique opportunities for cross-disciplinary skill development. | Scientific writing, Dynamic nature of science, and Information literacy Student appreciation of these skills may arise from GEOL’s mandatory senior thesis requirement. |
MATH | Working in groups, memorization, application to everyday life, dynamic nature of science | The student body is diverse (many are double majors, they move in and out of the major, there are multiple major tracks), making it challenging for instructors. There are limited opportunities for students to participate in research with mathematics faculty. | Working in groups Some faculty expressed frustration and recounted bad experiences, while others offered suggestions for more effective use. Memorization Some felt the K-12 trend towards de-emphasizing memorization has hindered student learning of more complex topics. |
PHYS | Scientific writing, working in groups, memorization, decision-making based on evidence | Faculty were disappointed that students had relatively little appreciation for working in groups. | Scientific writing Faculty believe it is important, but often do not know how best to teach it or who should teach it. Working in groups There was consensus that this was important to incorporate in classes. |
4.1 Intervention Planning Process
We assumed that every department held regular faculty meetings with high attendance, and anticipated that it would be straightforward to visit during one of these existing meetings. We emailed each chair individually asking for permission to participate in an upcoming faculty meeting. Reflective of how each department has its own norms and values, responses from chairs to our message varied. For example, the chair of the Math department shared that the department did not hold regular meetings of all faculty, in part because a high percentage were adjunct faculty. Instead, the department periodically convened a subset of faculty appropriate to the business at hand. He offered to meet with us along with the department’s undergraduate director to discuss our proposed presentation, and afterwards we held a special meeting for faculty members interested in teaching and learning.
Other chairs similarly worked with us to ensure strong participation. For example, the Computer Science chair was immediately supportive of the intervention and offered to provide lunch to help motivate attendance. The Physics chair invited us to a meeting where other important topics were scheduled to be discussed and voted upon, which he thought would encourage faculty member attendance; we did find that this meeting had the largest number of attendees. These experiences emphasize the importance of working collaboratively and flexibly with departments to maximize participation while respecting departmental norms.
4.2 Faculty Insight into Student Values
4.2.1 General Impressions and Major Discussion Points
Faculty meeting discussions were generally enthusiastic and robust, and filled the available time. The discussions were characterized by a great deal of interaction between faculty members, with minimal prompting from the facilitators (see example in Figure 1). Faculty expressed feelings ranging from pride (e.g., Chemistry faculty were proud to see that their students valued scientific writing skills highly) to disappointment (e.g., Physics and Biology faculty members were disappointed with the relatively low percentage of their students who valued scientific writing). Reactions to student values data differed across departments. For instance, most departments were proud that the majority of their students devalued memorization, but Math faculty almost unanimously were disappointed that this was the case. They expressed frustration about how memorization has become de-emphasized in K-12 schooling, leaving college students lacking in the basic knowledge needed to develop more advanced skills.
Several departments had extensive discussions about scientific writing and the potential impact of classroom experiences on student values. Chemistry faculty members explained that most of their courses incorporate writing assignments, and they believed that this encouraged students to value writing skills. Biology and Physics faculty members attributed their students’ low appreciation for writing to the fact that writing was rarely taught within courses in the major. Biology faculty members described a strong desire to require more written assignments, but felt that the grading volume would be insurmountable due to large class sizes. They also noted that national disciplinary standards in undergraduate biology emphasize writing to a lesser extent than in chemistry. Physics faculty members reported that they rarely taught writing because they perceived themselves as unskilled in teaching it, viewing it as a task better suited to the English department.
Faculty member 1: I feel that working in groups is something that the students actually hate. I don’t think it’s good training for a work place, at all. I think it’s a completely different thing to work together with my colleagues here than to solve a problem in class or give a group presentation.
TLC Director: It’s important to design a group assignment that requires each student to contribute. If you give an assignment that one individual can solve by himself or herself, there is nothing to gain from group [work].
Faculty member 2: There is some research evidence that shows that working in teams, for example, people who are coding in teams do so much better than if they are not in teams.
Faculty member 3: There are different ways to work in groups. It’s not only these big, formal problems. It could be something so simple as a think-pair-share activity. It could range from that up until a semester long project.
Faculty member 4: Could you create a website that students could go to where they could learn ways to be an effective group member?
TLC Staff Member: The campus general education group has put together a lot of resources for faculty for forming groups and evaluating groups, and for structuring assignments. There is also a really nice student-centered resource that is all videos, called [Surviving] the zombie apocalypse [at the University of] Minnesota, which was designed to prepare students for being part of a group.
Figure 1: Excerpt of Discussion in Entomology about Group Work Activities
4.2.2 Discussion of Student Demographic Characteristics
Faculty members also discussed the potential influence of individual student attributes on values. Geology and AOSC faculty members explained that their students appreciated skills used in research (e.g., scientific writing, communication) because they all were required to write a senior thesis. Astronomy, Computer Science and Math faculty members noted that their students were often double majors, so their responses were likely impacted by coursework outside the major, even though the prompt asked them to focus only on their major courses. Math faculty members mentioned that there were three main tracks within the major (statistics, theoretical math, and applied math), and speculated that values might differ between tracks. Faculty members also discussed the potential role of career aspirations. For instance, Biology faculty members reported witnessing a lower appreciation for research-related skills among pre-med students, who anticipate doing little future research. Similarly, Computer Science faculty members explained that their graduating students might devalue research skills because students are more likely to immediately enter the workforce rather than attend graduate school.
4.2.3 Suggestions for Survey Modifications
Math and Computer Science faculty speculated that some survey items, particularly those that included the word science, may have felt irrelevant to their students, and they suggested the survey items use more inclusive wording. Computer Science faculty members suggested adding an item on computational skills due to their high importance in computer science. Quantitative skills such as programming, statistical analysis, and using mathematical software were mentioned frequently in multiple departments. We used this feedback to subsequently revise and extend the STEP-U.
4.3 Faculty Values and Predictions of Student Values
Several differences between departments in faculty values were evident (Table 2). For instance, in most departments, fewer than half of the faculty members rated memorization as “important” or “very important,” but Math faculty members unanimously found memorization important. All departments devalued working in groups except for Computer Science, where 93% of faculty members rated it as important.
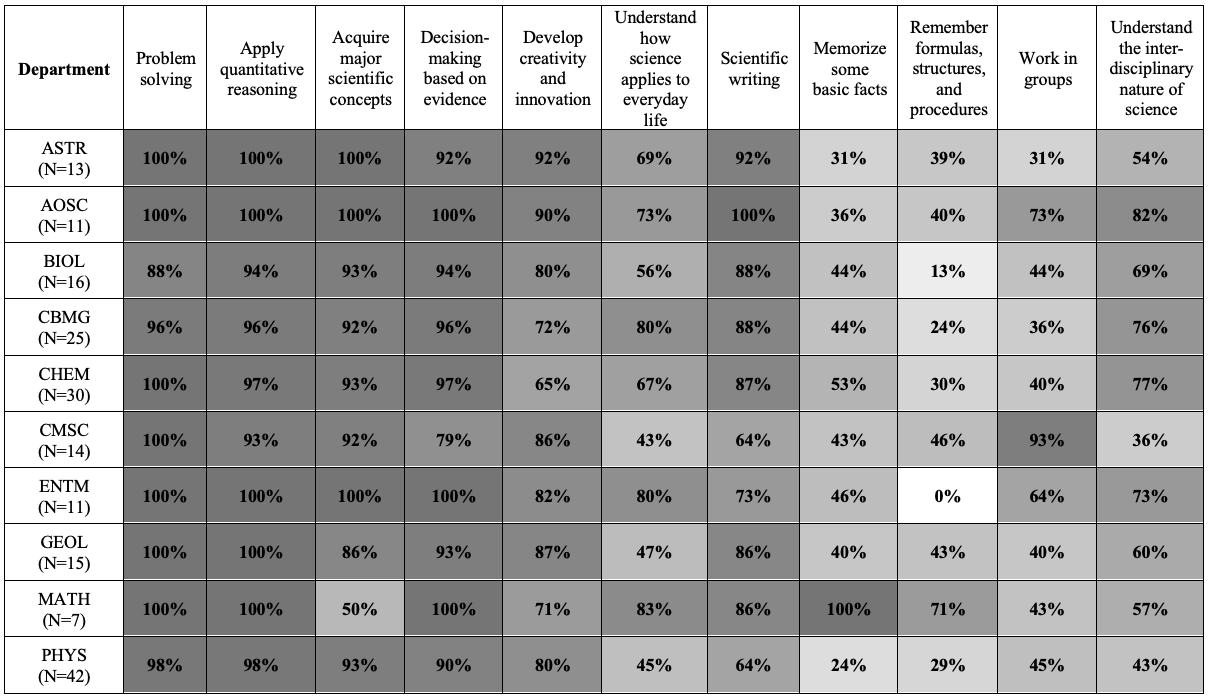
Note: Faculty members were asked to rate the importance of each skill for their students to acquire during their undergraduate studies on a scale of 1 to 5 (1 = not important, 2 = slightly important, 3 = fairly important, 4 = important, and 5 = very important). Percentages reflect the proportion of students giving ratings of 4 or 5 on the item. Darker cell colors indicate higher percentages of respondents rating the item as “important” or “very important.”
Faculty member predictions of how their students would rate each skill were also quite different from how the students actually responded. Table 3 provides an example juxtaposing faculty values, faculty predictions of student values, and student responses from the Biological Sciences (degree program offered jointly by BIOL, ENTM, and CBMG). The pattern in most other departments was similar, with faculty members largely underestimating the value that students attributed to cross-disciplinary skills. On observing their student results, one Chemistry faculty member said, “I’m kind of impressed that student priorities are similar to my answers and better than what I thought they would answer.” This correspondence between student and faculty values may reflect a disciplinary acculturation process, during which student values come to align with faculty values (Marbach-Ad et al., 2019).
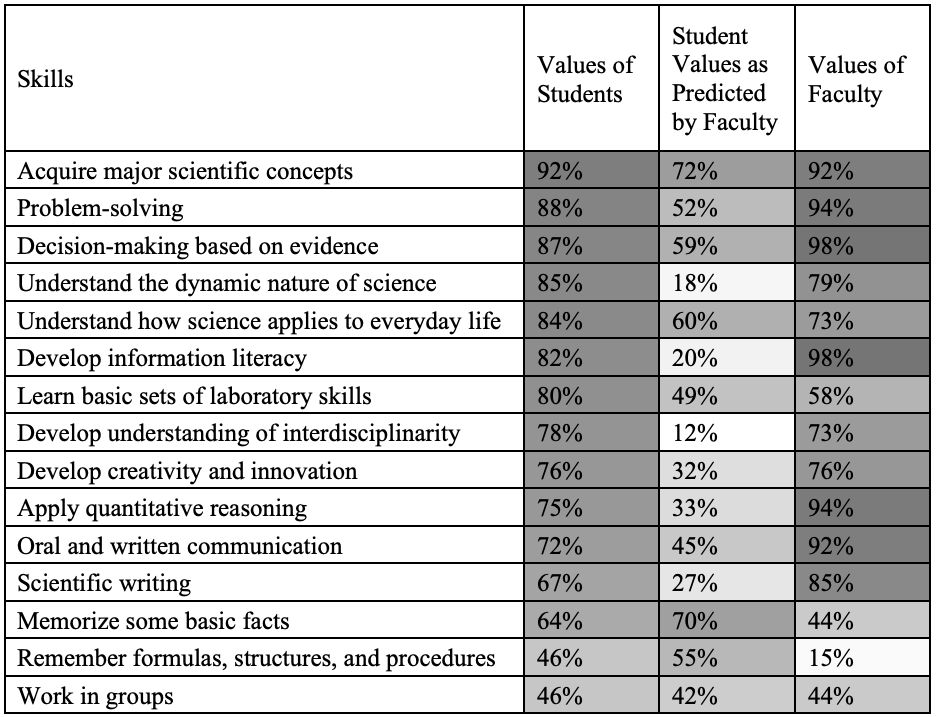
Note: We grouped the departments of CBMG, ENTM and BIOL, which collectively sponsor the biological sciences major (BSCI).
Darker cell colors indicate higher percentages of respondents rating the item as “important” or “very important.”
5 Implications for Teaching Professional Development
We used student data as a lever to engage faculty members in discussions about teaching by appealing to their identities as researchers (Brownell & Tanner, 2012; Robert & Carlsen, 2017; Savker & Lokere, 2010). This intervention differs from standard TPD interventions in that it gives faculty members the agency to interpret data from their own students and engage in conversation with their peers within the context of their department. We believe that this approach has the potential to inspire faculty commitment to enact change. As conceptualized by social cognitive researchers (Bandura, 1997; Pajares, 1992), thoughts and beliefs have strong influences on behavior. Given that these conversations encouraged faculty members to examine their beliefs and consider beliefs held by fellow faculty members, we anticipate that these conversations may ultimately lead to new departmental teaching norms and changes in teaching behavior. This prediction remains to be tested empirically.
This approach provided access to departmental faculty meetings in a way that facilitated department-wide discussions about teaching. Numerous conversations focusing on improvement of teaching occurred organically in the meetings. These conversations were strengthened by peer-to-peer interaction, whereby faculty members learned about specific student-centered approaches used by their departmental colleagues. The meetings also gave TLC staff new information about the unique needs and perspectives of each department, which will be used to inform future TPD. We were cognizant that, as representatives of the TLC, we might be perceived as outsiders who failed to understand disciplinary and departmental contexts; thus, we focused on facilitating the conditions for faculty members to self-generate knowledge and interpretations about student experiences. As these discussions progressed, we were often asked to suggest resources for effective teaching (e.g., Figure A1).
Our novel approach leverages professional identity within a disciplinary context to promote change in teaching. In our experience, when faculty members are asked to interpret locally-derived data (rather than reviewing data collected and summarized by external researchers), they show engagement, reflect deeply on their own teaching practices, and gain new insight into the student experience. These discussions expose faculty to effective teaching practices being employed by their peers, and we anticipate that this ultimately might inspire some to change their teaching. This strategy is consistent with the “middle out” model of institutional change in teaching (Bradforth et al., 2015) and is widely adaptable across disciplines and institutions.
6 Acknowledgments
This work was supported in part by a Howard Hughes Medical Institution Undergraduate Science Education Program grant to the University of Maryland. This work has been approved by the Institutional Review Board (IRB protocols #375954-8 and 404045-9). We thank the faculty members and students who participated in this study.
7 About the Authors
Gili Marbach-Ad is the Director of the Teaching and Learning Center (TLC) in the College of Computer, Mathematical, and Natural Sciences at the University of Maryland.
Carly Hunt is a research fellow in the Johns Hopkins School of Medicine and former graduate assistant within the College of Computer, Mathematical, and Natural Sciences at the University of Maryland.
Katerina V. Thompson is the Assistant Dean for Science Education Initiatives in the College of Computer, Mathematical, and Natural Sciences at the University of Maryland.
8 References
AAMC-HHMI Scientific Foundation for Future Physicians. (2009). AAMC/HHMI committee defines scientific competencies for future physicians. Howard Hughes Medical Institute. http://www.hhmi.org/grants/sffp.html
American Association for the Advancement of Science. (2011). Vision and change in undergraduate biology education: A call to action. AAAS. https://live-visionandchange.pantheonsite.io/wp-content/uploads/2013/11/aaas-VISchange-web1113.pdf
American Association for the Advancement of Science. (2015). Vision and change in undergraduate biology education: Chronicling change, inspiring the future. AAAS. https://live-visionandchange.pantheonsite.io/wp-content/uploads/2015/07/VISchange2015_webFin.pdf
Andrews, S. E., Keating, J., Corbo, J. C., Gammon, M., Reinholz, D. L., & Finkelstein, N. (this volume). “Transforming teaching evaluation in disciplines: A model and case study of departmental change.” In K. White, A. Beach, N. Finkelstein, C. Henderson, S. Simkins, L. Slakey, M. Stains, G. Weaver, & L. Whitehead (Eds.), Transforming Institutions: Accelerating Systemic Change in Higher Education (ch. 13). Pressbooks.
Andrews, T. C., Conaway, E. P., Zhao, J., & Dolan, E. L. (2016). Colleagues as change agents: How department networks and opinion leaders influence teaching at a single research university. CBE—Life Sciences Education, 15(2), ar15. https://doi.org/10.1187/cbe.15-08-0170
Anderson, W. A., Banerjee, U., Drennan, C. L., Elgin, S. C. R., Epstein, I. R., Handelsman, J., Hatfull, G., Losick, R., O’Dowd, D. K., Olivera, B. M., Strobel, S. A., Walker, G. C., & Warner, I. M. (2011). Changing the culture of science education at research universities. Science, 331(6014), 152–153. https://doi.org/10.1126/science.1198280
Austin, A. E. (2011). Promoting evidence-based change in undergraduate science education. National Academies National Research Council Board on Science Education. http://sites.nationalacademies.org/cs/groups/dbassesite/documents/webpage/dbasse_072578.pdf
Bandura, A. (1997). Self-efficacy: The exercise of control. Freeman.
Bradforth, S. E., Miller, E. R., Dichtel, W. R., Leibovich, A. K., Feig, A. L., Martin, D., Bjorkman, K. S., Schultz, Z. D., & Smith, T. L. (2015). University Learning: Improve undergraduate science education. Nature, 523(7560), 282–284. https://doi.org/10.1038/523282a
Bok, D. (2006). Our underachieving colleges: A candid look at how much students learn and why they should be learning more. Princeton University Press.
Brownell, S. E., & Tanner, K. D. (2012). Barriers to faculty pedagogical change: Lack of training, time, incentives, and… tensions with professional identity? CBE—Life Sciences Education, 11(4), 339–346. https://doi.org/10.1187/cbe.12-09-0163
Coppola, B. P., & Krajcik, J. S. (2013). Discipline‐centered post‐secondary science education research: Understanding university level science learning. Journal of Research in Science Teaching, 50(6), 627–638. https://doi.org/10.1002/tea.21099
Coppola, B. P., & Krajcik, J. S. (2014). Discipline‐centered post‐secondary science education research: Distinctive targets, challenges and opportunities. Journal of Research in Science Teaching, 51(6), 679–693. https://doi.org/10.1002/tea.21165
Corbo, J. C., Reinholz, D. L., Dancy, M. H., Deetz, S., & Finkelstein, N. (2016). Framework for transforming departmental culture to support educational innovation. Physical Review—Physics Education Research, 12(1), 010113. https://doi.org/10.1103/PhysRevPhysEducRes.12.010113
Desimone, L. M. (2009). Improving impact studies of teachers’ professional development: Toward better conceptualizations and measures. Educational researcher, 38(3), 181–199. https://doi.org/10.3102/0013189X08331140
Donald, J. G. (1997). Improving the environment for learning: Academic leaders talk about what works. Jossey-Bass.
Fairweather, J. (2008). Linking evidence and promising practices in science, technology, engineering, and mathematics (STEM) undergraduate education. The National Academies National Research Council Board of Science Education. https://otl.wayne.edu/wider/linking_evidence–fairweather.pdf
Finch, D. J., Hamilton, L. K., Baldwin, R., & Zehner, M. (2013). An exploratory study of factors affecting undergraduate employability. Education and Training, 55(7), 681–704. https://doi.org/10.1108/ET-07-2012-0077
Handelsman, J., Ebert-May, D., Beichner, R., Bruns, P., Chang, A., DeHaan, R., Gentile, J., Lauffer, S., Steward J., Tilghman, S. M., & Wood, W. B. (2004). Scientific teaching. Science, 304(5670), 521–522. http://www.jstor.org/stable/3836701
Hart Research Associates (2015). Falling short? College learning and career success. Selected findings from online surveys of employers and college students conducted on behalf of the Association of American Colleges & Universities. Hart Research Associates. https://www.aacu.org/sites/default/files/files/LEAP/2015employerstudentsurvey.pdf
Hart Research Associates. (2018). Fulfilling the American dream: Liberal education and the future of work. Selected findings from online surveys of business executives and hiring managers conducted on behalf of the Association of American Colleges and Universities. Hart Research Associates. https://www.aacu.org/sites/default/files/files/LEAP/2018EmployerResearchReport.pdf
Hativa, N. (1995). The department-wide approach to improving faculty instruction in higher-education: Qualitative evaluation. Research in Higher Education, 36(4), 377–413. https://doi.org/10.1007/BF02207904
Henderson, C., & Dancy, M. H. (2011, February). Increasing the impact and diffusion of STEM education innovations. National Academy of Engineering. http://www.nae.edu/File.aspx?id-36304
Heron, P., McNeil, L., Arion, D., Buell, W., Gates, S. M., Giri, S., McCormack, E., Quinn, H., Williams, Q., & Woolf, L. (2017). Phys21: Preparing physics students for 21st-century careers. American Physical Society. https://www.compadre.org/JTUPP/docs/J-Tupp_Report.pdf
Hora, M. T. (2016). Beyond the skills gap: Preparing college students for life and work. Harvard Education Press.
Jang, H. (2016). Identifying 21st century STEM competencies using workplace data. Journal of Science Education and Technology, 25(2), 284–301. https://doi.org/10.1007/s10956-015-9593-1
Kezar, A. J. (2001). Understanding and facilitating organizational change in the 21st century: Recent research and conceptualizations. ASHE-ERIC Higher Education Report, 28(4), 1–162.
Kezar A. (2013). How colleges change. Routledge.
Kezar, A. (2014). Higher education change and social networks: A review of research. The Journal of Higher Education, 85(1), 91–125. https://doi.org/10.1353/jhe.2014.0003
Marbach-Ad, G., Hunt, C., & Thompson, K. V. (2019). Exploring the values undergraduate students attribute to cross-disciplinary skills needed for the workplace: An analysis of five STEM disciplines. Journal of Science Education and Technology, 28(8), 1–18. https://doi.org/10.1007/s10956-019-09778-8
Matusovich, H. M., Paretti, M. C., McNair, L. D., & Hixson, C. (2014). Faculty motivation: A gateway to transforming engineering education. Journal of Engineering Education, 103(2), 302–330. https://doi.org/10.1002/jee.20044
National Research Council (2012). Discipline-based education research: Understanding and improving learning in undergraduate science and engineering. The National Academies. https://doi.org/10.17226/13362
Pajares, M. F. (1992). Teachers’ beliefs and educational research: Cleaning up a messy construct. Review of educational research, 62(3), 307–332. https://doi.org/10.3102/00346543062003307
President’s Council of Advisors for Science and Technology. (2012). Engage to excel: Producing one million additional college graduates with degrees in science, technology, engineering, and mathematics. President’s Council of Advisors on Science and Technology. https://files.eric.ed.gov/fulltext/ED541511.pdf
Quardokus, K., & Henderson, C. (2015). Promoting instructional change: Using social network analysis to understand the informal structure of academic departments. Higher Education, 70(3), 315–335. https://doi.org/10.1007/s10734-014-9831-0
Robert, J., & Carlsen, W. S. (2017). Teaching and research at a large university: Case studies of science professors. Journal of Research in Science Teaching, 54(7), 937–960. https://doi.org/10.1002/tea.21392
Rosenberg, S., Heimler, R., & Morote, E. (2012). Basic employability skills: A triangular design approach. Education and Training, 54(1), 7–20. https://doi.org/10.1108/00400911211198869
Savkar, V., & Lokere, J. (2010). Time to decide: The ambivalence of the world of science toward education. Nature Education.
Seymour, E. (2001). Tracking the process of change in US undergraduate education in science, mathematics, engineering, and technology. Science Education, 86(1), 79–105. https://doi.org/10.1002/sce.1044
Smart, J. C., & Ethington, C. A. (1995). Disciplinary and institutional differences in undergraduate education goals. New Directions for Teaching and Learning, 64, 49–57. https://doi.org/10.1002/tl.37219956408
Wieman, C., Perkins, K., & Gilbert, S. (2010). Transforming science education at large research universities: A case study in progress. Change: The Magazine of Higher Learning, 42(2), 6–14. https://doi.org/10.1080/00091380903563035
9 Appendices
9.1 The TLC Professional Development Activities
Appendix 1: The TLC Professional Development Activities
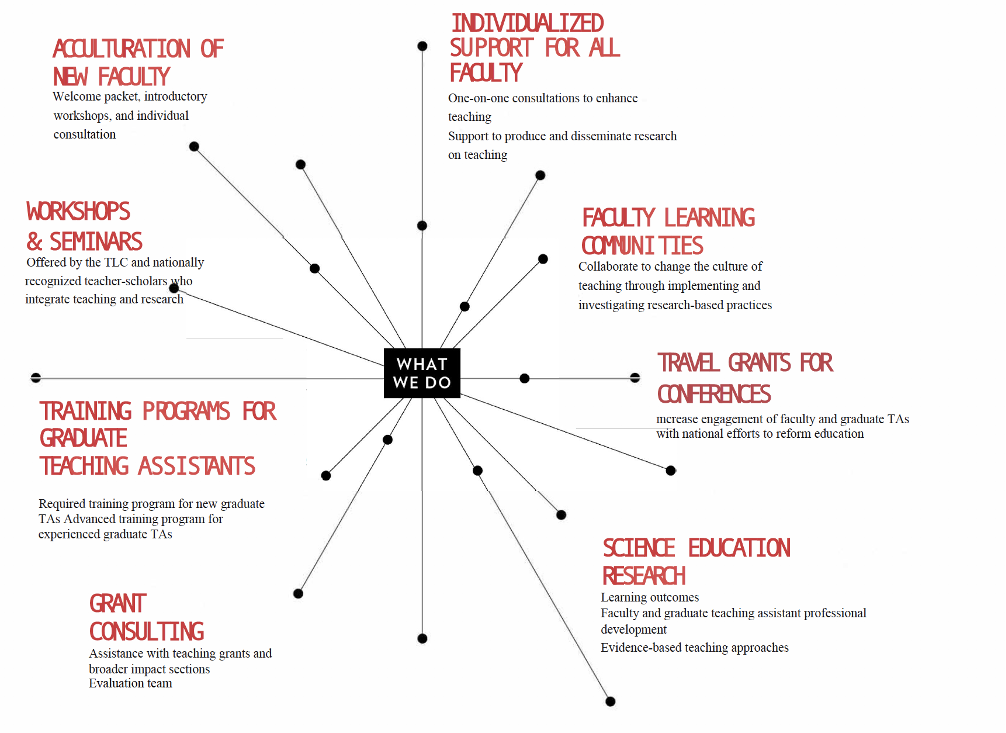
Appendix 2: Two-Page Handout Distributed During Computer Science Faculty Meeting (for more detail on the origins of these data see Marbach-Ad et al., 2019)
Demographic Characteristic | Computer Science | Mathematics | Biological Sciences | Chemistry | Physics |
Nsurvey (Ntotal_graduated) | 373 (894) | 195 (566) | 1389 (2571) | 260 (485) | 107 (294) |
Response Rate | 42% | 34% | 54% | 54% | 36% |
Gender (% Female) | 13% | 41% | 62% | 49% | 15% |
Underrepresented Minority (URM) | 9% | 7% | 13% | 12% | 8% |
Research Experience* | 27% | 29% | 63% | 68% | 69% |
Cumulative GPA | 3.2 | 3.3 | 3.4 | 3.4 | 3.2 |
Postgraduate Plans** | 47% | 64% | 89% | 80% | 79% |
*Percentage of students with on- or off-campus research experience
**Percentage of students intending to enroll in an advanced degree program in the immediate or near future
*** Samples for Biology and Chemistry include students graduating from 2011 through 2015. Physics, Computer Science and Mathematics samples include students graduating from 2012-2015.
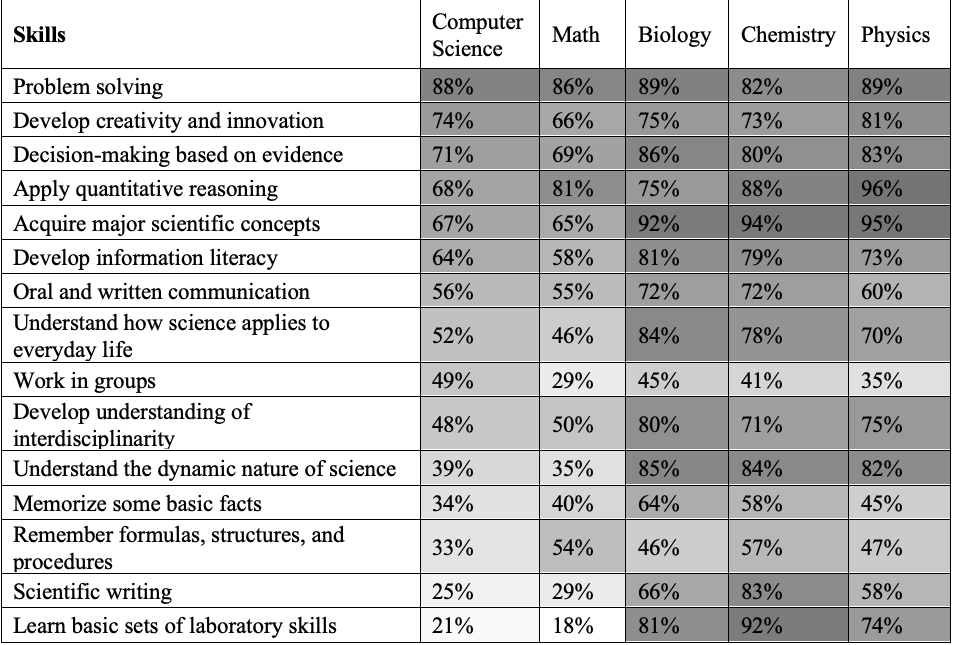
Findings from Student Surveys and Interviews
Problem Solving (Rated as “important” or “very important”: 88%)
Students felt this was the essence of computer science
Develop Creativity and Innovation (Rated as “important” or “very important”: 74%)
Students indicated that this skill was highly important, particularly for solving problems
Decision-making Based on Evidence (Rated as “important” or “very important”: 71%)
Students with prior research experience valued this skill to a greater extent than students who lacked research experience
Underrepresented minority (URM) students valued this skill more highly than non-URM students
Understand How Science Applies to Everyday Life (Rated as “important” or “very important”: 52%; Reported encountering this in some, most, or all major courses: 71%)
Students intending to pursue advanced degrees placed a higher value on relating course material to the real world than those not intending to pursue advanced degrees
Real world applications were encountered more frequently in upper-level courses
Work in Groups (Rated as “important” or “very important”: 49%; Reported encountering this in some, most, or all major courses: 49%)
Interviewed students thought that this skill would be important for their future careers in the computer science industry
Students reported that they experienced group work primarily in upper-level courses
Understand the Dynamic Nature of Science (Rated as “important” or “very important”: 39%)
Students with prior research experience valued this skill more highly than students who lacked research experience
Interviewed students expressed that this understanding is very important in computer science, as new algorithms, languages are constantly being developed
Students struggled with figuring out how this item related to their major because it included the word “science”
Memorize Some Basic Facts (Rated as “important” or “very important”: 34%) and Acquire Major Scientific Concepts (Rated as “important” or “very important”: 67%)
URM students placed a higher value on memorization relative to non-URM students
Students indicated that memorization was of low importance because basic facts can be looked up
Remember Formulas, Structures, and Procedures (Rated as “important” or “very important”: 33%)
Students with higher GPAs placed a lower value on this skill relative to those with lower GPAs
Students perceived this as important for exams, but not the real-world practice of computer science
Scientific Writing (Rated as “important” or “very important”: 25%; Reported encountering this in some, most, or all major courses: 32%)
Students drew distinctions between technical writing and scientific writing. Some felt that scientific writing would be important mainly for students seeking research-oriented careers.